Quantum simulators are setups which are designed to mimic the interactions and dynamics of other relevant many-body systems whose behaviour is very difficult to understand with current numerical methods. When such interactions are obtained in a continuous and global way, one talks about analog quantum simulators, which are generally more resilient to noise and easier to implement.
Among the different implementations, atomic and molecular optical (AMO) systems are some the most prominent examples of such simulators with many experimental realizations which have already benchmarked the validity of the quantum simulation approach.
In our group, we work intensively in these systems either by finding novel functionalities of these systems (e.g., simulating other problems), discovering new ways of detecting their properties, as well as developing new platforms that overcome the limitations of existing systems.
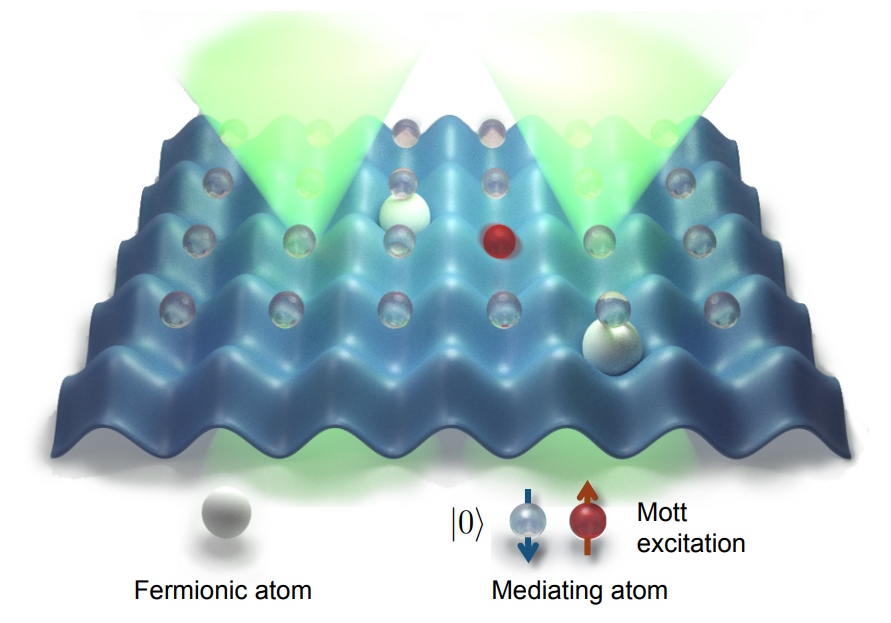
Ultra-cold atom simulators
Cold atoms in optical lattices are one of the most versatile analog simulators, which can simulate models in one, two, and three-dimensions. In these systems, the atoms are trapped by an optical potential made by sending counter-propagating lasers in free-space, such that both the atomic tunneling and interactions can be controlled to a large extent through these lasers. Originally, these systems were conceived as a way of simulating condensed-matter problems, such as electron transport in solids. However, in the recent years there is an interest in using them for different areas of physics such as high-energy physics or quantum chemistry.
In our group, we study the possibility of using these systems to emulate quantum chemistry dynamics [1] or non-trivial quantum optical phenomena with matter-waves [2]. Besides, we also develop new techniques to probe their emergent dynamics in, e.g., topological models [3].
Trapped ions simulators
Trapped ions are charged atoms that can be held in vacuum with electromagnetic fields. They form beautiful Coulomb crystals and they can be manipulated and addressed one-by-one with laser light or magnetic fields. That is the reason why trapped ions are the building blocks of one of the most promising technological platforms for quantum computing. But it turns out that this degree of controllability can also be used for quantum simulation. By shining a trapped ion crystal with laser light we can induce interactions between ions and make them emulate condensed matter systems such as quantum magnets, ultracold boson fluids, quantum Hall systems or tiny thermal machines.
In our group, we have made pioneering proposals in these systems to use them for simulating quantum magnets [4] or out-of-equilibrium dynamics [5]. In one of our collaborations with a Tobias Schaetz’ experimental group at U. Freiburg, we were able to investigate how thermal states emerge in a few ion crystal. More recently, in collaboration with the same group, we where able to manipulate the phonons in a trapped ion system, so that they would behave as electrons in a magnetic field [6].
New platforms for quantum simulation
Despite the excellent features of the two previously mentioned simulators, they also suffer from some limitations. For example, the atoms in optical lattices have limited kinetic energy as they hop through the lattice, setting stringent temperature requirements of the experiment. Besides, atomic interactions are generally local or short range.
For this reason, in our group we also develop alternative implementations that can overcome some of these limitations, like hybrid atom-nanophotonics platforms [7].
Selected Group Publications
- J. Argüello-Luengo, A González-Tudela, T Shi, P Zoller, JI Cirac. Analogue Quantum Chemistry Simulation. Nature 574 (7777), 215-218 (2019)
- I. de Vega, D Porras, JI Cirac. Matter-wave emission in optical lattices: Single particle and collective effects. Physical Review Letters 101 (26), 26040 (2008).
- A. Rubio-García, C. N. Self, J.J. García-Ripoll, and J. K. Pachos. Seeing topological edge and bulk currents in time-of-flight images. Phys. Rev. B 102, 041123 (R) (2020)
- D. Porras, J. I. Cirac. Effective quantum spin systems with trapped ions. Physical Review Letters 92 (20), 207901 (2004)
- G Clos, D Porras, U Warring, T Schaetz. Time-resolved observation of thermalization in an isolated quantum system. Physical Review Letters 117 (17), 170401 (2016)
- Floquet-Engineered Vibrational Dynamics in a Two-Dimensional Array of Trapped Ions. P. Kiefer, F. Hakelberg, M. Wittemer, A. Bermúdez, D. Porras, U. Warring, T. Schaetz. Physical Review Letters 113, 213605 (2019).
- DE Chang, JS Douglas, A. González-Tudela, C-L Hung, HJ Kimble. Colloquium: Quantum matter built from nanoscopic lattices of atoms and photons. Reviews of Modern Physics 90 (3), 031002 (2018)